Prescriptive Basement Wall Design
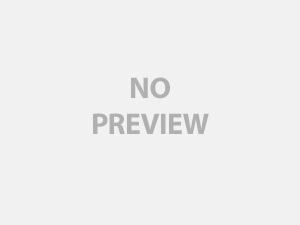
Outline
- Introduction
- Prescriptive Design
- Vertical Reinforcement Example
- Horizontal Reinforcement
- Connections at the Top and Bottom of the Wall
- Floor / Building Aspect Ratio
- Other Prescriptive Solutions
- Conclusion
- References
1. Introduction
The context of this article is the design of reinforced concrete basement retaining walls. By `retaining’ I mean that they retain (hold back) significant heights of backfill, tending to push inward on the basement of the structure. With as many basement walls as there are constructed in this country it is surprising how little design information is available on the topic. Indeed, pick any dozen reinforced concrete design textbooks, and the word `basement’ won’t even be in the index. A lot of engineers are designing basement walls, having learned basically by themselves, but are often too busy to write about it. This article aims to fill that gap and give some guidance to those in or who will be in the basement wall design business. I also include relatively new provisions in the model building codes that have bridged the gap (a little) in a prescriptive senses.
2. Prescriptive Design
Prescriptive design of basement walls essentially follows two main paths. First, for example, the International Building Code (IBC), Chapter 1805, provides prescriptive basement wall design for walls that are supported at the top (by a floor diaphragm) and at the bottom (being doweled into a footing). These provisions basically give the required amount of vertical reinforcement to resist various lateral pressures, the lateral pressures based on soil type. The presumed behavior of the basement wall is one-way, acting as a vertical beam pinned top and bottom, with beam lengthy equal to the height of the wall, and very wide (the length of the wall). No explicit benefit is given to support at returns, or bump-outs, and the walls are essentially allowed to be very long. The vertical pinned-pinned scenario is one in which the soil provides a triangular load on the wall and it flexes (bends) inward. The tension face is thus on the interior (living side) face, and thus that is where the reinforcing steel is detailed. The footing is assumed to not provide rotational resistance, and this is (presumed to be) designed with regard to resisting gravity loads (foundation self weight and superstructural gravity loads). Reinforcement requirements are given for various `unbalanced backfill’ heights reflecting the amount of backfill being retained.
Table 1805.5 (1) provides minimum wall thickness for plain concrete and plain masonry walls, and will not be discussed further. Tables 1805.5 (2,3,4) provide vertical reinforcement requirements for 8-in., 10-in., and 12-in. wall thicknesses, respectively, based on unbalanced backfill and three groupings of soil types (and presumed lateral pressures). Headings on the Tables dictate reinforcement location, and text of Section 1805.5 dictates grade of reinforcement, minimum concrete strength, use of alternate (size) reinforcement, etc. Soil types are per the Unified Soils Classification System.
3. Vertical Reinforcement
Determination of the vertical reinforcement will be illustrated by example.
Example.
Find: the reinforcement requirements for a 9-ft tall, 10-in thick wall with differential backfill height of 8 ft and with backfill material predominantly silty sand.
Solution: silty sand is `SM’ from the Unified Soil Classification System (or Table 1610.1 of the IBC, Chapter 16). From Table 1805.5(3) the required reinforcement is #6 @ 48 in. o.c. The header for the table requires that the reinforcement be placed at d âÂ?Â¥ 6.75 in. where d is the distance from the soil side (face) of the concrete to the center of the reinforcement (essentially the `effective depth’ in reinforced concrete design). Section 1805.5.2 requires that the reinforcement shall have a minimum specified yield strength of 60,000 psi, and that the concrete have a minimum compressive strength (28-days) of 2500 psi.
Section 1805.5.3 allows alternate reinforcement bar sizes, provided the spacings of the alternate bar sizes give an equivalent cross sectional area of steel (and are not spaced farther apart than 72 in.). For example, if we consider using #5 bar, the required spacing would be (and I’ll provide a simple formula),
… s #5 = s #6 (as #5 / as#6) = 48 in. (0.31 in.2/0.44 in.2) = 33.8 in. … use 32 in.,
where s refers to spacing and as is the cross section area of a single bar of the noted size.
The equation derives from equating the reinforcement `ratio’ of that derived from the Table value with the ratio of the alternate bars size and required spacing,
ÃÂ = as / (s d ) alternate values = as / (s d) Table values.
The above assumes a constant effective depth d in the conversion. We could tweak the d and thus the s, but that goes beyond the provisions of that section of the code and would thus require `engineering’.
So, an acceptable prescriptive solution for the vertical reinforcement would be …
… V.S.: # 5 @ 32 in. o.c. placed d = 6.75 in. from the soil (outside) face.
Discussion: I generally consider a basement wall to be a `wall’ with respect to ACI 318 (American Concrete Institute Building Code Requirements for Structural Concrete), Chapter 14, and thus never space the steel farther than 18 in. o.c. If, indeed, we specify 18 in. o.c. for the vertical reinforcement, we will find that # 4 bar will satisfy the minimum requirement, thus,
… V.S.: # 4 @ 18 in. o.c. placed d = 6.75 in. from the soil (outside) face.
Check: … s #4 = s #6 (as #4 / as#6) = 48 in. (0.20 in.2/0.44 in.2) = 21.8 in. … use … 18 in.,
In general I require 3000 psi concrete strength for basement walls, for the more extreme exposure conditions where I practice, which fulfills the 2500 psi minimum.
4. Horizontal Reinforcement
For horizontal reinforcement I generally use the temperature shrinkage minimum requirement of ACI, Chapter 7.12, or the minimum horizontal wall reinforcement, ACI 318 Section 14.3.3 (for above grade walls). Using ACI 318 Section 14.3.3 for our conditions would require ÃÂ min, h.s. = 0.0020. Such reinforcement would provide significant `horizontal’ or `two-way’ action, at least near returns, which has not been taken into consideration. (Thus, we are being conservative.) Alternately, temperature / shrinkage reinforcement from ACI 318 Chapter 7, would be ÃÂ min, t/s = 0.0018, not much difference. Depending on the edition of the code being used, the horizontal reinforcement requirements may be different, or absent. For example, two horizontal bars may be required at the top of the wall. In the absence of clear provisions or requirements, I use the minimum values of ACI 318 Chapter 14.
5. Connections at the Top and Bottom of the Wall
As noted, the prescriptive design thus covered assumes `pin’ connections at top and bottom. These connections resist `inward’ (horizontal) pushing, and for taller walls with significant unbalanced backfill, this `inward pushing’ is very significant. (And for a while the provisions to resist it were missing.)
At the Bottom
The bottom of the wall must be supported laterally by a `snug’ fit with the floor. A concrete slab is assumed. In this case the slab must not have expansion joints (at wall and throughout) and is assumed to bear against a wall at the opposite side of the basement (which bears against adjacent soil, and so on). Passive (side) earth pressure of the wall footing is not considered sufficient, unless, perhaps, the footing is deeply keyed into the supporting soil.
Top of the Wall
Previously mentioned is the required role of the floor to provide the pin connection at the top of the wall. `Conventional’ framing requirements are generally insufficient for common wall and differential backfill heights. Three elements must be considered: 1) the connection of the wall sill plate to the concrete wall; 2) the connection of the sill plate to the floor framing; and 3) the floor diaphragm overall.
Example
Consider a Residential basement wall height of 8 ft with differential backfill of 7 ft, clay soil. Determine the required anchorage of sill plate to the top of the wall and connection of framing to the sill plate. Use the International Residential Code (IRC) provisions.
Foundation walls are covered in Section R404 of the IRC. The IRC, also by the International Code Council, is often adopted for Residential Construction (One and Two Family Dwelling) kind of along side the IBC. In many cases there is strong overlap between the two (code), though not always. Generally the IBC may be used on all structures (including Residential), whereas the IRC is limited (to Residential).
The `symbol’ for clay soil is CL. This can be obtained from Table R401.4.1 (inferred) or from the Unified Soil Classification System (see References).
From Table R404.1(2) we obtain … Ã?½ in. anchor bolts @ 9 in. o.c. spacing.
Whoa! … that’s right! (If we do the `calcs’, we’ll essentially come up with the same thing.) That is a lot of bolts. And it is because there is a lot of pressure (theoretically). (Clays and silts are perhaps the worst for exerting lateral pressure.) The fact that more walls have `not failed’ in this regard is remarkable, probably due to the `plastic’ nature of the wood-frame with lots-of-nails construction, unaccounted for two-way-ness in the concrete walls, `factors of safety’, and, truthfully, that a lot of walls have failed (to some extent), and owners have lived with them anyway (or not).
For the sill plate to framing connection we obtain, from Table R404.1(1), …
… one Ã?¼ in. thick steel angle with the horizontal leg attached to the sill bolt adjacent to joist/blocking and the vertical leg attached to the joist or blocking with Ã?½ in. diameter bolt.
Wow; that is a lot of connection. The Table also gives the horizontal force being resisted (in this case 429 lb per foot). If approved by the Building Official, this number could be used to select connection devices to transfer the same load.
At this point we can’t help but look elsewhere in the Tables and see, for example, if our `soil’ had been `GW’, we could have `gotten by’ with Ã?½ in. bolts at 18 in. o.c., and a 20 gage clip with 5-8d nails per leg at each joist, and if we look at the force being resisted, it’s only 214 plf, instead of 429. (And, if we were also detailing the vertical reinforcement, we’d see a big difference also.) So, suffice it to say here, we may find it advantageous to haul away the clay soil, and bring in (well graded) gravel, for our backfill.
6. Floor / Building Aspect Ratio
Once the force at the top of the wall is delivered to the floor, the floor either acts as a big compression member taking it to the opposite wall, where the condition is mirrored, or the floor acts as a diaphragm (big, flat horizontal beam) and takes the load (out) to the side walls (the so-called daylight-basement). In this second case, instead of requiring that we `engineer’ the floor diaphragm, we are given aspect ratio limits for the structure. If, in the preceding example, we have the daylight basement scenario with the floor acting as a diaphragm, the maximum aspect ratio would be 1.1 for our CL soil. Thus, the length of the residence could be no more that 1.1 times the width. (If we backfill with GW the aspect ratio climbs to 2.1 for our scenario; the house can be twice as long. Obviously, these kind of issues are best wrestled with early in the design development process, as compared to later.)
7. Other Prescriptive Solutions
Some building authorities have adopted prescriptive solutions that may be far more relaxed on the wall-to-floor connection but may require shorter wall lengths or limited distances between returns (or jogs) in the wall. And, where the designer wants to `go past’ these limits, buttress or counterfort walls may be required. In these cases the walls act more like two-way slabs, with or without the floor at the top being depended on.
8. Conclusion
The prescriptive solutions described above (except under the `other’ category) assume one-way behavior, assume full lateral support at the top of the wall, and, except for the daylight basement scenario, may allow for long walls. Openings in the floor against the basement wall should not be planned, unless specific provisions are found that allow such. (Put the stairs elsewhere!) If you can’t make your project `fit’ the prescriptive provisions, you’ll need to come up with an `engineered’ solutions. In fact, in some jurisdictions (with lousy soils), you’ll need to have them engineered anyway. Also note: the prescriptive design calls for the steel to be placed toward the inside face of the wall, with presumably the horizontal reinforcement tied to it, and not in the wall center. While this is the most efficient for one-way wall behavior, it is not the most efficient for two-way wall action. We will see when we discuss two way behavior of basement walls it may be the best to place the reinforcement (back) at the center.
9. References
Building Code Requirements for Structural Concrete, ACI 318, American Concrete Institute, P.O. Box 9094, Farmington hills, Michigan, 48333.
International Building Code, International Code Council, 4051 West Flossmoor Road, Country Club Hills, IL 60478.
International Residential Code, International Code Council, 4051 West Flossmoor Road, Country Club Hills, IL 60478.