Reproductive Linkage Between Steatonyssus and Their Vespertilionid Hosts
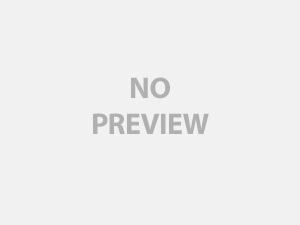
Much of the information on ectoparasites of mammals are documents their presence and abundance. Bat ectoparasites have perhaps received less attention than those of many other mammals, largely due to difficulty in acquiring the hosts. The ectoparasites of the big brown bat, Eptesicus fuscus (Beauvois 1796), have received more attention in the past (Whitaker and Wilson 1974) due to the abundance of their host and the bats habitat of roosting in or near manmade structures. To date, 31 species of ectoparasites have been reported for this species, with 17 of these being recovered in Indiana (Mumford & Whitaker 1982, Chapter 1). The evening bat, Nycticeius humeralis (Rafinesque 1818), has received significantly less attention, being more geographically restricted than big brown bats. Whitaker and Wilson (1974) reported one species of ectoparasitic mite (Steatonyssus ceratognathus) from N. humeralis, while Acanthophthirius nycticeius was added by Fain and Whitaker (1987) and Cimex adjunctus was added throughout its range (Ubelaker and Kunz 1971, Ritzi and Clark 2001, Sparks et al. 2003). Of these parasites, the macronyssid mites, Steatonyssus ceratognathus and S. occidentalis, are the most prevalent and abundant ectoparasites on evening and big brown bats respectively. Steatonyssus mites are typical macronyssids, in that they develop through the stages of egg, larvae, protonymph, deutonymph, and mature adult. Only the protonymph and adult forms feed (Radovsky 1967), and as such, are the only stages commonly encountered on the host. The other life stages are found in the roosts of the bats, where they have easy access to hosts after they molt into protonymph or adults, or are such short-lived that they are rarely recovered. Few studies have examined the biology of macronyssid mites (Radovsky 1967, Lavoipierre and Beck 1967, Beck 1966), and these have indicated that mite reproduction is linked to temperature and relative humidity. Beck (1966) also tested whether testosterone had an effect on reproduction, with negative results. However, the use of a male hormone may not have been appropriate to test on mite reproduction in light of the roosting ecology of the host. As female bats form large maternity colonies, and it is within these colonies that the majority of the mites associated with bats exist, a female hormone may have been a better choice.
Originally, parasitic interactions with host reproduction were believed to be connected with the process of female mate choice (Hamilton and Zuk 1982). The idea behind this was that females would choose a mate based on parasite resistance, which should indirectly imply “good genes”, while rejecting parasite ridden males. This hypothesis has been tested several times in avian studies, with females choosing mates not burdened with parasites (Moller 1993, Kose et al. 1999, Griffith 2000, Roulin et al. 2001, and Moore and Wilson 2002). Another addition to the hormone, parasite, reproduction triangle has been the creation of the immunocompentence hypothesis. Folstad and Karter (1992) stated that the expression of male secondary sexual characteristics and immunosuppression are regulated by parasite burden. Therefore, as testosterone stimulates development of secondary sexual characteristics, this same testosterone will suppress the male’s immune system and make him more susceptible to parasitic infection. Alternately, if one is combating parasites and having to maintain an elevated immune response, one may be less able to develop secondary sexual characteristics than one who is not parasitized. This theory has also been heavily tested in the avian literature (de Lope at al. 1998, Brinkhof et al. 1999, Poiani et al. 2000, Proctor and Owens 2000), and been found to support the trade-off of immune function and body condition created by parasites and androgens. The relationship between higher levels of testosterone in the host and higher parasitic infection has also been seen in other groups, such as with larger parasitic burdens on wild caught male Sceloporus undulatus compared to females, as well as higher levels on males artificially implanted with testosterone (Klukowski and Nelson 2001).
Immunocompetence does not just affect the adults; however, as the young maturing in the nest also must deal with the detrimental effects of parasites. In sand martins, nestlings had a higher survival rate when parasites were removed (Szep and Moller 2000). Similar effects have been seen in great tits (Richner at al. 1993) and cliff swallows (Brown and Brown 1986) in the negative impact of parasitism on fledgling body mass. Adults can counteract this by modification of nest ecology, such as reducing the size of colonies, choosing parasite-free nests, or by switching roosts to minimize exposure to parasites (Christe et al. 1994, Lewis 1995, Lewis 1996).
The ongoing evolutionary conflict between host and parasite may best be fought without struggle (Renaud and De Meeus 1991). The theory of reduced escalation of aggressiveness would indicate that parasites might modify their life patterns to maximize their fitness without detrimentally injuring the host, i.e. their environment (Nelson et al. 1975, Marshall 1981). One strategy toward minimizing negative effects is to synchronize host and parasite reproduction to take advantage of resources when they are most available. This was first documented in the rabbit flea and its mammalian host (Rothschild 1965, Rothschild et al. 1970), with fleas following the hormonal cycle of their reproductive hosts. Spilopsyllus cuniculi requires a blood meal containing corticosteroids in order to mature, and changes in these hormones affect maturation rate in this species. Similar situations have also been seen in lice on seals (Kim 1975), Orange-crowned warblers (Foster 1969), and dermanyssid mites on the European house sparrow (Phillips 1972). A recent study (Christe et al. 2000) on the bat Myotis myotis in has shown that the spinturnicid mite, Spinturnix myotis, also increases in abundance on female hosts during the reproductive cycle. This is the first published record of a reproductive linkage between a mesostigmatid mite and a chiropteran host, suggesting the possible reproductive linkage between the mite family Macronyssidae and their chiropteran hosts in the New World.
Nycticeius humeralis is believed to mate in late fall through early spring (Mumford and Whitaker 1982). Once the bats have left their hibernacula, the females migrate northward and form maternity colonies, while the males form independent bachelor colonies in the more southern extent of their range (Watkins 1972). The females stay in these colonies through pregnancy, lactation, and post-lactation until they depart for their winter hibernacula. The young follow the adult females to hibernacula within a few weeks, and then only females return once more in the spring to repeat the cycle. Eptesicus fuscus follows similar patterns, in that males and females hibernate together during winter, and then females form maternity colonies in buildings in which to rear their young (Mumford and Whitaker 1982). Males tend to either roost singly, sometimes a few in the same roost with females, or they may form small bachelor colonies (Barbour and Davis 1969). Taking the natural history of these animals into account, a male hormone may not have been as good a choice as a female hormone, such as estrogen or prolactin, at assessing changes in acarine populations in connection to host reproduction. A male hormone would be expected to have a greater impact on host-acarine interaction while male bats are reproductively active. However, as this coincides with hibernation, a mite that reproduced and demanded a great deal of energy from a torpid or semi-torpid host would seem to be a poorly co-evolved parasite. Therefore, this study focused on the seasonal changes in Steatonyssus abundance on female bats from maternity colonies over the course of pregnancy through post-lactation and consequential departure to the hibernacula.
Ectoparasites are unique in that they live on a living environment which can be considered hostile to their existence (Price 1980). Not only do they have to deal with interspecies competition, but they must also contend with their host’s attempts to conserve its own resources. As such, the reproduction and dispersal of a parasite must be timed to coincide with adequate resources and reduced host disturbance. For this reason, populations of ectoparasites may change greatly over both time and in their ratio of adults to protonymphs. Parasitic abundance can change from host to host, colony to colony, or from one time of the year to another on the same host. A combination of different factors could influence these changes, including ecology, morphology, physiology, or climate (Dick et al. 2003). For this reason, understanding more about which factors influence mite reproduction and dispersal is essential to understanding their life history. The objective of this study was to determine if changes in mite population were synchronized with host female reproductive state.
Methods and Materials
Bats were mist-netted along Prairie Creek, Vigo and Sullivan Counties, Indiana from May through October of 1999 through 2002; from the Indianapolis International Airport (IIA), Hendricks, Mario, and Morgan Counties, from May to September 2001 through 2003, and from Camp Atterbury Reserve Training Facility, Bartholomew, Brown, and Johnson Counties, from June through August 2002. Prairie Creek is a 650 hectare bottomland forest, in which bats were captured at seven netting stations approximately 0.5 kms apart. The IIA consists of many small, fragmented woodlands surrounded by agriculture, residential areas and industry (Sparks et al. 1998). Mist-netting took place at roughly 1 km distant sites along the East Fork of White Lick Creek between US Highway 40 in Indianapolis (Marion County), south near the city of Plainfield (Hendricks County) and to Mooresville (Morgan County). This work was conducted in an area under mitigation for the federally endangered Indiana bat, Myotis sodalis. Some netting efforts were also conducted in a woodland at IIA known to contain both a M. sodalis and N. humeralis colonies. Camp Atterbury Reserve Training Facility is largely undeveloped forest surrounded by fields and manmade structures (Whitaker and Gummer 2003). Mist-netting efforts at Atterbury were made along the various streams that fed into the Driftwood River, as well as across several small to medium-sized ponds. In an effort to not habituate the bats to the respective netting sites, netting stations were rotated so that collections were not conducted at any one site within 3 days. Most net sites consisted of a pair of two vertically-joined 2.5 X 9 m 50/2 38 mm mesh mist-nets (Avinet, Inc.) mounted on telescoping poles (Kunz and Kurta 1988), although most netting at Prairie Creek was conducted with only one double height net apparatus. Bats captured were removed from nets and immediately placed into individual cloth bags and tied to prevent contamination. Bats were identified to species, weighed, sex and reproductive condition assessed, and given an individually number aluminum wing band. Reproductive stage was determined as per Racey (1988) for palpating pregnant females, and expressing milk from lactating females. Juveniles were separated from adults by the presence of epiphyseal-diaphyseal gaps in a trans-illuminated wing (Anthony 1988).
Additional big brown bats were recovered by collecting from within building colonies and structures. The primary building colonies sampled were located in Prairie Creek, Vigo County (2 buildings); Plainfield, Hendricks County (1 building); and Tunnel Cave, Cliffy Falls State Park, Jefferson County (1 railway tunnel). Building colonies were sampled in order to obtain ectoparasite data from non-volant juveniles, for comparison with the parasitic loads of juveniles after volancy. The hibernacula at Tunnel Cave gave insight as to the winter population of ectoparasites associated with big brown bats. Only limited collections were made under these circumstances, to minimize disturbance to the bats. A more involved study of the wintering acarine fauna associated with bats in Indiana will be presented later.
Ectoparasitic examinations took place in the field by restraining the bats by the wrists with wings outstretched against a flat surface. Bats were visually scanned for parasites by examining the wing and tail membranes, head, ears, and fur. Fur examination was conducted by examining fur parted with dissecting needles and by combing with a metal flea comb. Parasites were recovered with the use of fine forceps and placed in vials of 70% ethanol. Bats were then examined using Jeweler’s glasses (10X) in the above manner to locate any parasites missed by the initial visual examination. The time allowed for each bat examination was not to exceed 20 minutes, and averaged 5-10 minutes per bat. Ectoparasite information recorded included identification of parasite to family, life stage and sex of acarine parasite, site of each parasite at first observation, and number of parasites at that location. Ectoparasites were returned to the laboratory where they were cleared and mounted on glass slides with PVA medium (Bioquip Products, Gardena, CA). Slides were then identified to species using Radovsky (1967) for macronyssid mites, Usinger (1960) for Cimex, and Whitaker (1982) for other ectoparasites. Voucher specimens of Steatonyssus ceratognathus and S. occidentalis have been deposited in the Indiana State University Vertebrate Collection Ectoparasite Depository (ISUVCED). All handling of bats was conducted under a state collecting permit and animal and care use protocol issued to the primary investigator (IACUC 01-CR).
Statistical analysis-Data were tested for homogeneity using a Levene’s test, and the data sets from both bat species violated the criteria of homogeneity and normality. Therefore, initial analyses were conducted using Kruscal Wallace nonparametric tests to infer if differences existed between parasite loads among the host reproductive stages. Upon evidence of differences, parasite count data were log transformed as per Krebs (1999), and run in one-way ANOVA’a and MANOVA’s with Tukey post-hoc tests to determine were differences in mite counts occurred between reproductive life stages. Statistical analysis was carried out according to Zar (1996), and performed using SPSS for Windows 11.5 (SPSS, Inc., Chicago, IL). Prevalence, mean intensity, and abundance were calculated as per Bush et al. (1997).
Results
A total of 435 individuals of Nycticeius humeralis was examined between the 1999 and 2003 field seasons, yielding a total of 26,909 ectoparasites. The ectoparasites recovered included Steatonyssus ceratognathus (n = 26,888), Cimex adjunctus (n = 16), Macronyssus crosbyi (n = 2), Ripiaspichia americana (n = 2), Myiatrombicula esoensis (n = 1), and Androlaelaps fahrenholzi (n = 1). Prevalence and mean intensity are presented for each species in Table 14. In comparison, 735 individuals of E. fuscus were examined during the same period and yielded a total of 15,870 ectoparasites. The ectoparasites recovered included Steatonyssus occidentalis (n = 15,616), Ornithodoros kelleyi (n = 100), Spinturnix bakeri (n = 67), Cimex adjunctus (n = 46), Nycteridopsylla chapini (n = 22), Acathophthirius caudatus (n = 9), Cryptonyssus delsultorius (n = 4), S. ceratognathus (n = 3), Macronyssus crosbyi (n = 2), and Euschoengastia pipistrelli (n = 1). Prevalence and mean intensity for each species is presented in Table 14.
Nycticeius humeralis-A breakdown of evening bats per reproductive stage produced four life stages with large enough sample size to analyze: pregnant females (n = 97), lactating females (n = 81), post-lactating females (n = 90), and volant juveniles (n = 164), for a total of 432 bats used in the analysis. Three non-reproductive females were excluded from analysis due to small sample size, and male and female juveniles were combined after tests showed differences to be nonsignificant (Krustal Wallace test, X2 = 0.01, p = 0.921).
Lactating hosts possessed the greatest abundance of total mites (x = 97.5), followed by juveniles (x = 74), post-lactating adults (x = 39.3), and pregnant bats (x = 33.9). A graphical representation of total mites by date helps to further illustrate that the peak occurs during lactation (Figure 4). When total mites were analyzed by adult female, adult males, and protonymphs, similar trends were seen in the adult female and protonymphs for lactating adults and juveniles, while numbers of adult males were too low to detect a difference between reproductive stages (Figure 5).
Log transformed data were then analyzed with an ANOVA for effect of host reproductive class (Table 15). Year was found not to produce a significant effect (F (df = 3) = 1.621, p = 0.199) for S. ceratognathus, and was excluded from further evening bat analyses. For total mites, lactating bats had significantly more parasites than any other reproductive stage, with juveniles, pregnant, and post-lactating adults forming an unresolved group. Within each life stage examined, significant differences in numbers recovered per host life stage were noticed. Adult female mites were more abundant on a group composed of lactating and pregnant hosts, followed by a pregnant and juvenile group, and last by a juveniles and post-lactating adult group. Adult male mites occurred in greatest numbers on pregnant hosts, with the least number recovered from juvenile bats. Protonymphs were significantly more abundant on lactating hosts than on the other reproductive stages, with a group composed of juveniles and post-lactating adults bearing more parasites than the post-lactating and pregnant adult group.
Eptesicus fuscus- A total of 733 big brown bats was utilized for analysis, after the removal of two non-reproductive females. There were six viable reproductive classes: pregnant (n = 71), lactating (n = 172), post-lactating (n = 83), juvenile female (n = 181), adult male (n = 109), and juvenile male (n = 117). Juvenile male and female bats were tested for differences and males were found to be distinct, having greater abundances of total mites (Kruscal Wallace X2 = 3.67, p = 0.056). Differences between mite life stages provided similar but non significant results between juvenile hosts using Kruscal Wallace tests (Adult female mites: X2 = 3.19, p = 0.074; Adult male mites: X2 = 0.008, p = 0.928; Protonymph mites: X2 = 3.75, p = 0.053). Due to the results of adult female and protonymph mites forming trends toward significance, juvenile male and female bats will be examined independently for this species of host.
I also examined for differences in parasitic abundance between volant and non-volant pups. Non-volant pups in the roost were found to have an abundance of 5.04 +/- 9.76 (n = 48), while volant pups collected later in the season had an average abundance of 12.24 +/- 28.75 total mites (n = 246). Testing these samples with an independent samples t-test resulted in a significant value (t (df = 294) = 3.17, p = 0.002), yielding a mean difference of 7.18 +/- 2.27 mites.
Lactating big brown bats hosted the highest abundance of total S. occidentalis (x = 43.2), followed by pregnant (x = 31.1), post-lactating adults and juvenile males (x = 15.0), adult males (x = 12.3), and juvenile females (x = 9.1) (Figure 6). After mites were broken into their three life-stage classes, similar trends were seen for the protonymphs. Adult female mites; however, had higher abundances on pregnant hosts (x = 4.5) than on lactating hosts (x = 4.2), while juvenile females (x = 1.1) harbored more mites than adult males (x = 0.8) (Figure 7). The remaining trends observed in S. ceratognathus were seen for the other S. occidentalis life-stage classes.
After log transforming the count data, ANOVA’s conducted on parasite abundance by host reproductive class showed a significant difference between host classes for total mites (F = 29.96, p < 0.001) (Table 16). Tukey post-hoc tests (Ã?± = 0.05) indicated that host classes grouped as follows in descending order of mite abundance: Lactating, Pregnant/Post-lactating, Post-lactating/Juvenile Male/Juvenile F, and Juvenile M/Juvenile F/ Adult Male. ANOVA's performed on the three mite life-stage categories indicated that protonymphs (F = 25.07, p = 0.001) and adult female mites (F = 17.05, p = 0.001) existed in greater abundances on lactating and lactating/pregnant hosts, respectively, while male mites showed no significant difference (F = 0.44, p = 0.821) (Table 16). Tukey post-hoc tests (Ã?± = 0.05) showed protonymphs to have basically the same groups as the total mites due to their high sample size, with the exception of juvenile males being included in the second largest abundant group with pregnant and post-lactating adults. Adult female mites differed in that lactating and pregnant hosts formed the group with the most abundant parasites, while juvenile males/post-lactating adults/juvenile females/adult males formed a single secondary group.
For big brown bats, a significance difference by year was detected for total mites (F = 2.40, p = 0.05) and protonymphs (F = 2.56, p = 0.04) using a MANOVA comparing reproductive class and year by log-transformed mite abundance. However, these results only successfully separate the numbers of mites collected in 1999 from 2003. Only 11 big brown bats were examined in 1999 during the preliminary analysis as compared to 63 through 360 hosts in following years. Therefore, effect of year is probably due to low sample size in 1999 skewing the data.
Discussion
Nycticeius humeralis – Evening bats had different abundances and mean intensities of S. ceratognathus on different reproductive stages of the host. In general, the total number of mites at the beginning of the host reproductive season occur at low levels (Figure 4). This supports earlier work (Radovsky 1967) that the mites tend to occur in low intensities in the cold inactive time of hibernation. After the bats have migrated to their maternity roosts, the mites begin to increase in numbers during pregnancy. This pattern continues through lactation, when mite levels peak on adult hosts. Abundances begin to decline on adult females during weaning and post-lactation, returning to pre-maternity season levels prior to preparation for hibernation. When pups became volant, they played host to the highest intensities of mites observed during the study, with some individual pups possessing over 600 mites. Within a month of volancy, however, pups begin to have mean intensities similar to post-lactating adults. This initial disparity between pups and adults is believed to be an artifact of the process of efficient grooming behavior. After pups are first born, it is understood that their mothers groom them as well as nurse them in the roost. Later, when the pups begin weaning, they are less efficient at the process of grooming then their mothers (McLean and Speakman 1997). It apparently takes time for them to master this skill and lower parasite levels to that of the previous generation.
Females and protonymphs showed trends similar to total mites, while the small numbers of adult male mites prohibited analysis. In particular, nymphs outnumbered adult female mites while infesting lactating and juvenile hosts. On lactating hosts, nymphs outnumbered adult female mites at 3.2:1, while nymphs occurred in lower intensities (2.3:1) compared to females on juvenile bats. This appears to represent the pattern of dispersal and infestation that occurs on juvenile bats. Large numbers of adult females (x = 13.4) have been observed on the dorsal uropatagium of lactating hosts, and then appear in high densities on juvenile hosts after this pattern is no longer seen (See Chapter 4). In addition, I observed protonymphal mites climbing onto the backs and clinging to adult mites during this time. As the adult mites are more mobile that nymphal mites, they may provide a mechanism in which dispersal is more readily available. Also, since the adults are more mobile, the presence of higher numbers of adult mites on juvenile bats is further explained, as the presence of gravid adult females increases the probability of a successful colonization of the new host over time.
The transformed data indicate that the period of lactation is indeed the time in the host’s reproductive cycle when mites reach their peak. Secondary periods of high infestation are pregnant and juvenile bats for adult female mites, while juvenile bats alone form a second tier of infestation for protonymphs. The lowest levels of infestations occur at the beginning and the end of the reproductive season, with post-lactating and pregnant hosts harboring the lowest levels of nymphs of all the reproductive classes. These results further illustrate the pattern of mite reproduction and abundance over the reproductive season of the evening bat.
Eptesicus fuscus – Similar results to that of evening bats occurred in big brown bats, with a few notable exceptions. Due to the differences in roosting habitats (E. fuscus occurring in less densely packed colonies in buildings compared to the tighter-packed colonies of evening bats in tree cavities), big brown bats harbor lower abundances of mites on average. Even with this difference in maternity colony structure; however, the increase in total mites through pregnancy and lactation, followed by the decline in post-lactation, still holds true. Post-lactating females had mite intensities not significantly different from adult males. This suggests that in the non-reproductive season, male and female bats would harbor comparable ectoparasitic burdens. Also, although juvenile bats were separated by sex due to differences near significance, the slightly higher levels of mites on males indicates that the differences between male and female bats may not be as strong a factor as in other animals during development.
As adult male bats co-occur within the distributional range of females during the maternity season, either within the maternity colony but apart from the females or in separate bachelor colonies, their parasite burdens provided a comparison between females and males over the same time period. These data support those of other studies (Moore and Wilson 2002) which indicate that although males of most groups tend to harbor higher parasite burdens, in the case of Chiroptera, the opposite appears to be true. Whether this is the result of colonial factors (such as number of individuals in the colony, microclimate of the roost, grooming efforts), a physiological effect, (hormone profiles, immunological responses), or larger body size is current unknown and deserves further study. The difference in mite abundances between the sexes does appear to be influenced by coloniality, as the opposite trend was seen in juvenile male and female pups possessing a near significant difference (Kruscal Wallace X2 = 3.67, p = 0.056), with male bats harboring more mites than females. This lends one to believe that all things equal, male bats may indeed have a higher parasitic burden than females. However, the structure and roosting characteristics of the bat community, with females possessing high population densities and greater opportunities as new hosts than males, creates a situation in which the patterns in mite numbers have evolved in the female maternity season.
Reproductive females show the greatest change in mite population structure, not just in total mites, but also in the life stages of S. occidentalis. The majority of adult female mites are found on lactating and pregnant bats, with secondary levels infesting the remaining host classes, with their peak on lactating bats. Protonymphal S. occidentalis occur in different groups of decreasing abundance, with the highest infestation on lactating bats. The remaining 3 groups generated by the Tukey post-hoc test indicate the difficulty in resolving the differences between the other reproductive classes. Although pregnant bats have more nymphs than the other classes, they are still clumped with post-lactating and juvenile male bats in the second group. This shows the higher levels of mites on juvenile males over their female counterparts. There are relatively few mites on juveniles of both sexes and adult males. Unlike evening bats, where juveniles supported incredibly high numbers of mites during early volancy, big brown bat pups did not show this phenomenon, but this appears to be an effect of the lower levels of mites associated with big brown bat colonies.
Pups prior to volancy were also examined. These observations showed that pups in the roost under maternal care possess fewer parasites than independent juveniles after weaning. Christe et al. (2000) showed that pups under maternal care have fewer spinturnicid mites than pups lacking maternal care, yet have levels of infestation comparable to their lactating parents. These findings show that although independent pups possess more macronyssids than their mothers while still under maternal care, they possess fewer mites than their mothers. This may illustrate one of the costs of maternal care, that mothers spend time grooming their suckling pups that they could spend caring for themselves. The cost of increased grooming has been shown to decrease body condition and health (Giorgi et al. 2001), thus lactating bats presumably only can afford to spend a limited amount of time grooming, and must therefore ration the time between self grooming and pup grooming. The trade-offs between current and future reproduction appear to be skewed in favor of current reproductive effort while the pups are nursing, and then shifted toward future reproduction while the pups are still in the roost but not dependant on milk. There were also observations of several pups that appeared to be abandoned in the roost that further support these findings. These pups, while still less than one month of age based on size, harbored massive parasitic loads of macronyssids (n = 129 mites) and cimicids (n = 8). The importance of maternal grooming therefore would seem valuable for protecting and ensuring the development of young bats.
The impact of ectoparasites has influenced the evolution of their hosts in various ways. Parasites have been implicated in changes in roost fidelity (Lewis 1995), as well as other natural history traits, such as reduced clutch size, reduction in mating success, decreased territoriality, and reduced birth weight (Forbes 1993, Moller 1993, Perrin et al. 1996). The synchronization of reproduction between parasite and host is another example of the influence of parasites on their hosts, as well as the adaptability of parasites to best utilize resources when they become available.
Literature Cited
Anthony, E. L. P. 1988. Age determination in bats. Pp. 47-58. In: Ecological and Behavioral Methods for the Study of Bats. T. H. Kunz, editor. Smithsonian Institutional Press. Washington D.C.533p.
Barbour , R. W. and W. H. Davis. 1969. Bats of . University Press Kentucky Lexington. 286p.
Beck, A. J. 1966. Factors affecting the density an occurrence of ectoparasites on bats. Unpublished Ph. D. Dissertation. University of California Davis. 97p.
Brinkhof, M. W. G., P. Heeb, M. Kolliker, and H. Richner. 1999. Immunocompetence of nestling great tits in relation to rearing environment and parentage. Proceedings of the Royal Society of London B 266: 2315-2322.
Brown, C. R. and M. B. Brown. 1986. Ectoparasitism as a cost of coloniality in cliff swallows (Hirundo pyrrhonota). Ecology 67: 1206-1218.
Bush, A. O., K. D. Lafferty, J. M. Lotz, and A. W. Shostak. 1997. Parasitology meets ecology on its own terms: Margolis et al. revisited. Journal of Parasitology 83: 575-583.
Christe, P., A. Oppliger, and H. Richner. 1994. Ectoparasite affects choice and use of roost sites in the great tit, Parus major. Animal Behaviour 47: 895-898.
Christe, P., R. Arlettax, and P. Vogel. 2000. Variation in intensity of a parasitic mite (Spinturnix myoti) in relation to the reproductive cycle and immunocompetence of its bat host (Myotis myotis). Ecology Letters 3: 207-212.
de Lope, F., A. P. Moller, and C. de la Cruz. 1998. Parasitism, immune response and reproductive success in the house martin Delichon urbica. Oecologia 114: 188-193.
Dick, C. W., M. R. Gannon, W. E. Little, and M. J. Patrick. 2003. Ectoparasite associations of bats from central Pennsylvania. Journal of Medical Entomology 40: 813-819.